In the late 1980s, Japanese biologist Yoshimori Ohsumi finally got to run a lab of his own and began casting around for a suitable topic to occupy himself and his new grad students. At 43 years old, he did not consider himself much of a scientific success; he was now hoping to corner a niche of biology to call his own and chose a relatively obscure topic in the biology of bakers’ yeast. A little less than three decades later, Ohsumi has been awarded the 2016 Nobel Prize for Physiology or Medicine for what his seemingly arcane research ultimately showed about human health, disease, and aging. Ohsumi’s story illustrates the essential role that fundamental research —science performed for the sake of understanding the natural world— plays in medical advances.
His insights revealed how our cells renew themselves. Nearly all the proteins in the human body are constantly destroyed and replaced in a carefully orchestrated garbage disposal and recycling scheme. Without this system, our cells would be quickly overwhelmed with damaged and potentially toxic junk. One of the most vital components of this cellular housekeeping is a process called autophagy, which means, in Greek, “self-eating.”
Before Ohsumi’s work, the importance of autophagy was not widely appreciated, and the mechanics of how a cell could dine on itself were unknown. In the 1950s, biologists discovered a compartment in the cell filled with degradative enzymes, an organelle they named the lysosome (for “digestive body”). The enzymes in the lysosome break down large biological molecules like proteins, carbohydrates, fats, nucleic acids, and membranes into their component parts. Through the microscope, scientists could see bubbles of doubled-up membranes—autophagosomes—delivering debris to the cell’s degradation center. But the details of how this system worked were unclear. How do the autophagosomes know which cell components should be delivered to the lysosome? How do they engulf and transport their targets? What other events in the cell depend on it? What happens when autophagy grinds to a halt?
These questions went unanswered because nobody knew the identity of genes specifically involved in autophagy. This was a roadblock because biologists rely heavily on the tools of genetic analysis when exploring new and unknown processes. Without an idea of the genes involved, they didn’t know what proteins took part in autophagy, or how to unambiguously identify autophagosomes, how to block autophagy, or how to quantify it. So for decades, the molecular details remained mysterious.
In 1988, Ohsumi decided to tackle this problem using the yeast Saccharomyces cerevisiae, the familiar fungal cells that help bakers to bake bread, brewers to brew beer, and winemakers to ferment wine. Yeast also frequently help biologists to make knowledge. They are popular in the lab because they are single cells—so they are considerably easier to study than multi-celled organisms—but they still have the complex internal organization common to plants, animals, and fungi. They are also much easier to genetically manipulate than many organisms. In effect, they provide scientists with a fast-growing, inexpensive, powerful, safe, and mostly pleasant-smelling alternative to studying our own cells.
Yeast had rescued Ohsumi from his lab struggles during an often frustrating postdoctoral stint in the United States. Defeated by an initial project on in vitro fertilization in mice, he had switched to studying how yeast cells duplicate their genome during cell division. When he moved back to Tokyo in 1977 to the lab of Yasuhiro Anraku, Ohsumi continued with his new study subject, but worked on transport systems that moved small molecules like amino acids and calcium into and out of the yeast version of the lysosome (idiosyncratically known by yeast biologists as the vacuole—which means “empty space”).
Once he became an associate professor, Ohsumi needed stake out new territory for his own lab. He decided to explore how the vacuole breaks down biomolecules. He had a simple but powerful plan to test whether autophagy occurred in yeast: he examined the vacuoles of yeast deficient in key enzymes that degrade proteins. If autophagic vesicles were being delivered to the vacuole but their degradation was blocked, they should start to build up.
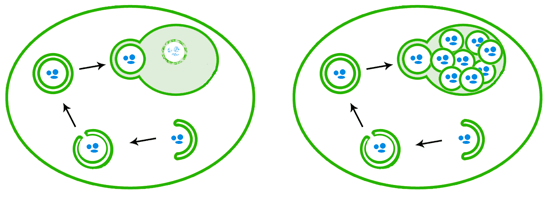
When autophagy is induced, a double layer of membranes wraps around cellular debris, eventually sealing up its target inside a closed autophagosome. The autophagosome then fuses with the lysosome/vacuole, releasing a single-membrane bound package that is then degraded by enzymes (left). In vacuole degradation mutants like those used in Ohsumi’s lab (right), the autophagosome contents that are delivered to the vacuole are not degraded and instead accumulate.
Knowing that autophagy in animal cells was stimulated by starvation, he grew some of his mutant yeast in growth medium that was nutritionally deficient, and he then examined the cells under a simple light microscope. Within an hour, a few tiny wobbling blobs appeared within the vacuoles. Within three hours, the vacuoles were massively bloated and so jam-packed with the spherical blobs that they could no longer wobble. These structures were the remnants of autophagosomes that had been delivered to the vacuole. In normal cells, they would be rapidly degraded by the vacuolar enzymes, but in the mutants, they just kept arriving at the vacuole and were never broken down. The autophagy traffic was piling up.
Ohsumi and his students had not only shown that yeast cells underwent autophagy, they now had a means for finding the genes that controlled it. Grad student Miki Tsukuda embarked on a mission to find other yeast mutations that affected autophagy. She treated the protein-degradation deficient strain with a chemical that induces random mutations, then screened these mutated strains in a two-step procedure: she first isolated all the strains that had trouble surviving starvation conditions, then she examined their vacuoles under the microscope to hone in on those with autophagy defects. In these effective but labor-intensive experiments, Tsukuda found 15 different genes that disrupted autophagy when mutated. She had uncovered most of the building blocks of autophagy.
Once Ohsumi’s group and others learned the location and sequence of the mutated genes, they had the challenging job of figuring out what job each gene performed in the autophagic pathway. Intriguingly and at first unhelpfully, most of the proteins produced by these genes were new to science, which meant the scientists could not rely much on studies of other biological pathways as a guide. In spite of this, the elegant mechanisms that drive autophagy were gradually revealed, and robust methods to study the process in other organisms were developed. It soon became clear that autophagy in yeast was very similar to the process in animals, including humans. The field exploded. The year Ohsumi and Tsukuda published their yeast screen, only a couple of dozen research papers were published on autophagy. So far in 2016 alone, nearly 4,000 have been published.
Part of the reason for the field’s growth is the importance of autophagy for health and disease. The ability to recycle nutrients and cellular components helps our cells to survive starvation. Autophagy is important during the development of embryos. It is involved in fighting infection by bacteria and viruses. When autophagy stops functioning, the buildup of toxic proteins can lead to neurodegenerative diseases. When autophagy goes into overdrive, it is associated with cancer formation. With such a key role in maintenance of the cell, many other disease links are being uncovered every year, and there is intense interest in developing drugs that can influence autophagy.
Ohsumi and his students, postdocs, and collaborators have had an outsized influence on this field, not only in identifying the genes that underlie cellular recycling, but in revealing many of the molecular details of how these players function. By seeking out unexplored territory in our understanding of the cells that make bread rise, yeast biologists provided a map for understanding ourselves.